Pic Programming Guide Beginners
We've put it off for long enough - it's time to jump in the water! We are going to use Microchip assembler for this. People are often afraid of assembly language, but for a simple processor like the PIC, it's really not too bad. The programs you'll write will generally be quite small, and provided you comment your code properly, you should find that it's quite straightforward. We first need to consider the 'programming cycle'.
The first step is to write the source code, and save it as an.ASM file. Next using MPASM, you assemble the source code. This will create a number of files, the most relevant being the list file (.LST) and, if you're lucky, the hex file (.HEX). If there are any errors encountered during the assembly process, there will be no.HEX file, and you'll need to examine the.LST file and correct the errors in your.ASM file.
The next step is to open the.HEX file with your programming software, and actually program the PIC. Then, place the PIC into the test circuit, and see if it works. Invariably, you'll have to go back to the.ASM file and make some more modifications to the source code. This cycle repeats until the PIC does what you require.
You can download an IDE (integrated development environment) from Microchip called MPLAP - this brings together the first few steps of the process. This sounds like a good idea, but earlier versions of MPLAP were somewhat clunky, and I quickly found that it was better to use ConTEXT, which can be configured to act a bit like an IDE. During this section, I'm assuming that you've installed and configured ConTEXT as described on this page. Having said that, MPLAP is much better these days, and is worth investigating.
It incorporates an emulator, which allows you to step through your programme on a line-by-line basis. This is useful at the beginning, but when you start interfacing with real hardware like LCD displays, this becomes less useful. Programme specification and flowcharts: At the risk of stating the obvious, we must decide what we actually want our programme to do. Expressing this in simple words is the first step.
4 Bit Counter: A program to count from 0 to 15, and output the result in binary on four ports. The counter should increase by 1 (increment) every second. When the counter reaches 15, it should reset and continue counting from 0. The next step to to convert these words into a flow diagram. If you've done programming before, you were probably advised to use flow diagrams, but, if you were like me you probably didn't see the point - after all, high level languages like BASIC are so easy to understand that by the time you've draw the flowchart, you might as well have written the code! But, right at the very start, we must accept that we need to use some sort of tool to understand the process.
Believe me, you do need flowcharts! Start by drawing up a simple flowchart. This is a good start, but the next stage is to zoom in and add some detail to the counter section: With this flowchart, we've introduced the idea of decision making. By following through the diagram, you should be able to see how we start by resetting the counter, and increase it until it gets to the highest value required. Then the counter is reset and the whole cycle repeats. But there are still some sections missing.
We haven't incorporated a means of outputting the counter value. Without this, our PIC will be merrily counting away to itself, but we'd have no idea if it was working or not. The exact position of the output stage could appear in one of two places, but the key thing is to ensure that it is within the main counter loop. If it was placed after the 'reset counter' box, but before the arrow coming from the decision box, then we would only ever see zero on the output. By putting the output box before the 'increase counter' box, the counter will actually start from zero.
If placed after the increase, then the first number outputted will be '1'. But with this increase in the detail, we need to have a careful think about our algorithm. Following it through from the beginning, we can see that the counter will start at zero, this number will be output, then it will be increased by one, and as it doesn't yet equal 15, this '1' will be outputted. Follow this through, and you should see how the counter will happily increase in value, outputting the result as it goes. But, we have a problem here.
Think about it before scrolling down. What happens when the counter gets to 15?
Work around the loop, assuming that the counter has just been increased to 14. This '14' is outputted, and is then incremented to equal '15'. But, as we get to the decision box, the programme realises that the counter has reached 15, and it will take the alternative path and the counter will be reset. We never get to see '15'! The solution to this is simple: change the fixed number in the decision box to 16. This will be included in the next revision of the diagram, along with the final thing - the delay.
Without it, the counter will count far to quickly for us to see it. So we need to add the delay, but where? Like the output box, it must be within the 'inner' loop. How about making it the first item in the loop? This will work, but think about what happens the very first time the program runs - the output won't be updated until after the first delay routine. We don't know what happens when the PIC is initially powered up, so it safest to take control as soon as we can. This flowchart incorporates the correction to the counter and the time delay.
Also, I've added a section called 'initialise hardware', which is an essential step in any PIC programme. Comparing this flowchart to our initial attempt, you can see that we've 'drilled down' and added considerable detail. This simple process is applicable to any programming challenge, no matter how large and complex, and it is well worth getting used to it right at the beginning. These sketches are very simple to make on paper - it's worth saying that the more time you spend away from the computer at first, the quicker your programme will work! Coding: So, we've got to a stage where we can begin translating those boxes into lines of code. Knowing when you can make this step comes with experience, but when a lot of the boxes are describable with single lines of code, you know that you're nearly there.
We'll start with ' Reset counter'. There are two ways to do this: movlw d'0';Place 0 into working register movwf Count;Move W into Count The first statement moves a literal number into the working register. A literal is a number that is fixed when the program is assembled, and cannot be changed as the program runs. So this line will always place zero into the working register, and to change this you will need to reassemble and re-program the PIC. The second line moves the working register into a file register. We discuss this in more detail later, but for now, assume that the Count variable has been previously set up. Note also that comments follow the semi-colon.
This is perhaps the logical and obvious method. Remember that the working register is the equivalent to the Accumulator in other processors, and most operations work through it. This is fine, and will work.
But it occupies 2 memory locations, and as the 16F84 only has 1024 words available in programme memory, it pays to think about code-efficiency right from the beginning. You can do this using only one instruction: clrf Count;Reset Count This instruction clea rs a file register. Clearly, in this context, means set to zero.
So, this rather handy instruction achieves the same as the above two lines in half the space. As an added bonus, it doesn't touch or affect the working register in any way. Next, we need to ' Output Count'. Assume that all of PORTB has been set up to be outputs. You'll remember from before that PORTB appears in the memory map, and as far as the processor is concerned, it's just RAM. So we can write to PORTB in the same way as we wrote to Count above. Movfw Count;Move Count into W movwf PORTB;Write W to PORTB The first line copies the value stored in Count to the working register.
The next line writes it to PORTB. If there are LED's connected to PORTB, they will light up to show a binary representation of the value of count. Suppose Count was 15, then four LED's would light as '1111' is 15 in binary.
A good understanding of binary and hex number systems is useful for any sort of 'machine code' programming. The next box on our flow diagram is ' Wait 1 second'. For the purposes of this introduction, we'll use some code that has been written before - we'll examine it later. But it's a good excuse to introduce the concept of subroutines. A subroutine is a section of code that has deliberately been placed outside of the normal program flow.
But, your main program can jump to the subroutine whenever it needs to - and at the end of the subroutine, the program flow will return to where the subroutine was called from. Subroutines are extremely useful, and we'll talk in more detail about them later. So, we just need one line: call Wait1Second;Call the 1 second subroutine Think of this as 'modular programming' - the subroutine can be copied and pasted from another program. Next is ' Increment counter'. As is often the case, there is more than one way to do this. You might have spotted the ADDLW instruction on the quick-reference sheet. Movfw Count;Move Count into W addlw d'1';W = W + 1 movwf Count;Count = Count + 1 This is perfectly reasonable, and is the most logical.
But there are variations on the theme: movlw d'1';W = 1 addwf Count,f;Count = Count + 1 This occupies one less programme memory location, and introduces instructions that have a destination in their syntax. Have a look at the quick-ref sheet: addwf FileReg, dest People often see the 'dest' part of the instruction, and think that 'dest' can be any file register they like.
Unfortunately, it can only be F or W - in other words, the result of the operation will be placed back into the file register, or left in the working register. This is something to check for if your programme doesn't behave as expected.
Also, if you omit the destination, the assembler won't generate an error - rather it puts a warning in the.LST file, which is easy to miss. In that instance, it assumes F - which obviously will work if that is what you wanted in the first place! There's an even easier way: incf Count,f;Count = Count + 1 This instruction increments the file register, and note that you have to specify the destination - in this case, we want the result to go back into the file register. Hopefully, everything has been reasonably logical so far. But now, we need to decide if the counter has reached 16, and this is where things get confusing! There are only 4 instructions that deal with making decisions, and they all work by testing individual bits in a file.
The trick is to make use of the various status flags that are available in the processor - refer to the STATUS register on page 2 of the quick-reference. The Z flag is set whenever the PIC performs an arithmetic operation - have a look at the third column of the instruction set sheet, and you'll see that some instructions affect Z, others don't.
The easiest way to see if two numbers are equal is to exclusive-or them. This almost certainly needs some explanation, so let's start with the truth-table for an exclusive-OR gate. 0 Look at this result - when number A and B are the same, the result is zero. This is an important principle, which we shall exploit next. Consider these lines: movlw d'16';W = 16 xorwf Count,w;W = 16 XOR Count The first line simply puts 16 into the working register.
Remember, this is a constant value, and if you decided that your counter needed to count up to some other number, you would have to re-programme the PIC. The second line does the XOR - but note what happens to the result - it is left in the working register.
It would be a disaster to put it into the file register, because the result from the sum is absolutely meaningless. As mentioned above, if you neglected to type the ',W' after ' Count', the assembler would assume a destination of 'F', and the programme would appear to count in a random sequence! So, we've effectively 'thrown away' the result from the XOR. But the key thing is that this line has affected the Z flag. If the two numbers are the same, then the result will be zero. If the result from an operation is zero, then the Z flag will be set.
And we can test for this: btfss STATUS,Z;Check the Z flag in the STATUS register;Is it set? Goto Loop; No, so Count 16 - keep counting goto Reset; Yes, so Count = 16 - reset counter The first line here performs a bit test on a file register, and will skip the next instruction if the bit is set. So, if the Z flag is clear, the program just flows onto the next line, and meets the goto Loop instruction. If the Z flag is set, the first goto is skipped, and the programme flow is diverted to the goto Reset instruction.
All of this needs a little thinking about, but it will make sense eventually. It might seem complicated because of the number of steps that we have to make, especially as everything else has seemed so straightforward up to now. It's worth mentioning that famous law of engineering - if there is a 50% chance of getting something wrong, you will! This applies here because there is a sister instruction - btfsc which means bit test on a file, skip if clear. Using this instruction reverses the logic, so you would need to swap the order of the goto statements to make this work. So let's bring together all of the lines that we've got so far: Reset clrf Count;Reset Count Loop movfw Count;Move Count into W movwf PORTB;Write W to PORTB call Wait1Second;Call the 1 second subroutine incf Count,f;Count = Count + 1 movlw d'16';W = 16 xorwf Count,w;W = 16 XOR Count btfss STATUS,Z;Check the Z flag in the STATUS register;Is it set? Goto Loop; No, so Count 16 - keep counting goto Reset; Yes, so Count = 16 - reset counter You see that I've added the labels that correspond to the two goto statements - compare this to the flow diagram and don't continue until you understand it!
Unfortunately, you can't just type all of that into a text editor and expect it to work. The assembler needs much more information before it can create a.HEX file. For example, it doesn't know which PIC processor you wish to use. It won't understand 'Count', or 'Wait1Second'.
So, how do we turn this into a working programme? The best way to start is with a standard template.
This is split into a number of sections:. Program name and comments.
Assembler directives. Memory definition. Main program. Subroutines Looking in detail at each of these: 1. Program name and comments: This really is just comments for your own use, and can take any form you like. My advice is to be as explicit as you can and include as much information as you can think of - assume that when you look back on your code in a few months time, you will have forgotten everything! Assembler directives: This is where we tell the assembler what processor we're using, and set other environment options.
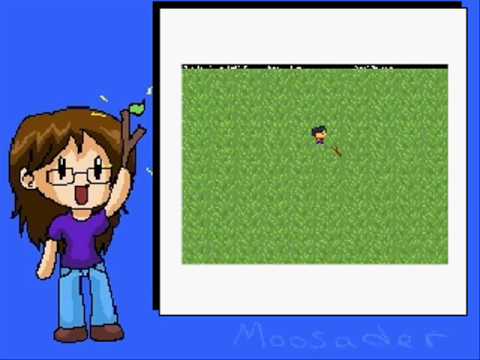
Let's look at an example: LIST P=16F84, R=DEC FUSES XTOSC & WDTOFF & CPOFF & PWRTEON include 'P16F84.inc' The LIST statement tells the assembler to switch on output to the.LST file. Next, the processor is defined as a PIC16F84, and the default radix is set to decimal. You might have noticed that we were writing numbers as d'16', for example.
Should you want to enter a binary number, you can write b'00001111'. Should you wish to use hexadecimal, you can write h'0F'.
This ability to use any number system you wish is really convenient. The default radix setting tells the assembler how to interpret any numbers that it finds without the letter and quotes. This is the most useful setting, but you can change this here. This is something that hasn't been mentioned yet, but fuses are a separate section of memory that is outside the normal programme memory.
These vary from device to device, and the datasheet for the PIC you wish to use will explain more fully, but basically options like oscillator type and code protection are set up here. Finally, the correct.INC file is included. This line simply tells the assembler to find the appropriate file, and insert it into the.ASM file at this point.
These include-files are supplied by Microchip as part of the assembler, and simply tell the assembler what numbers it should assign to words like STATUS and PORTB. Take a look at the memory-map on the PIC quick-reference sheet, and you'll see that PORTB lives at memory location 6. This might be in a different location for another PIC, so that's why the separate include file is used. It's worth saying that you can write your own include files that contain your own sub-routines. Personally I prefer to copy and paste in the subroutines are needed, as you can see everything on one screen, but when programs get bigger and more complex, this might be a useful technique.
Memory definition: The next thing is to list all the variables that your programme wants to use - this pre-warns the the assembler of all the words that is likely to come across. This can cause some confusion at first, but the key thing to remember that you just deciding where in memory you are storing your variables. Look again at the memory map, and you'll see that the first free GPR (general purpose register) is 0Ch (that's 12 in decimal) Ram EQU h'0C' Count EQU Ram+0 nextvariable EQU Ram+1 anothervar EQU Ram+2 There's lots of ways of doing this, but this is how I tend to lay them out.
Should I write a program, and decide to 'port' it to another processor, then I simply need to change the number on the first line. For example, the first free address on a PIC16F877 is 20h (32 decimal) 4. Main programme: That's the bit that we've already written! Subroutines: As we said earlier, subroutines need to be away from the normal program flow.
After the end of the main programme code is the logical place, but it's really up to you. 0 It might surprise you to see that the assembler turns these two different instructions into exactly the same machine code - 0086h.
This is the number that is actually programmed into programme memory, and when the core of the process meets this, it knows that it should load the contents of memory location 06h into the working register. While 06h is the correct address for PORTB, 86h is the address of TRISB. Just to be completely explicit, compare the binary representation of these two numbers: 7 6 5 4 3 2 1 0 PORTB 06h 0 0 0 0 0 1 1 0 TRISB 86h 1 0 0 0 0 1 1 0 As you can see, the difference is bit 7. And as the previous table shows, there isn't space to store bit 7 in the programme memory along with the instruction. To get around this problem, the designers at Microchip decided to store this 7th bit somewhere else - in the STATUS register. C There are lots of other things in here - you've already met the Z flag.
But we're interested in something called RP0, which is our 8th bit. You might notice RP1 - this is the 9th bit for even bigger PICs - you won't need to worry about this until you graduate to bigger processors like the PIC16F877 that I used in the hi-fi preamp. This tells us that in theory, a PIC can have up to 512 locations in RAM. Note that STATUS register is available on both sides of the memory map. As above, compare the addresses given for STATUS: 7 6 5 4 3 2 1 0 STATUS 03h 0 0 0 0 0 0 1 1 STATUS 83h 1 0 0 0 0 0 1 1 Again, the only difference is the MSB (most significant bit).
This duplication of STATUS in both sides of the memory map is absolutely essential! Imagine you've set RP0 to move to Bank 1. What happens if STATus was not in Bank 1? You would never be able to access STATUS again, meaning you could never change back to Bank 0!
So this background hopefully explains what RP0 is, and why we need to set it. If it doesn't make complete sense at this stage, don't worry too much. But do try to revisit it soon, because it is an essential topic to understand. Meanwhile, back to the programme: It's time to introduce two more instructions. PIC processors offer lots of bit-oriented instructions - that is, instructions that operate on just a single bit within a file register. That's convenient for setting and clearing RP0 - let's look at the initialisation subroutine again:;.Init - set up all ports, make unused ports outputs Init clrf PORTA;all of porta low clrf PORTB;all of portb low bsf STATUS, RP0;change to bank1 clrf TRISA;all of porta outputs clrf TRISB;all of portb outputs bcf STATUS, RP0;back to bank0 return Note the ' bsf' instruction - this means set a bit in a file register. Refer to the quick-ref sheet and you'll see that the syntax of this is: bsf FileReg, bit So, if you had an LED connected to bit 0 of PORTB (RP0 for short), you could light that LED with bsf PORTB,0.
There is a complimentary instruction of bcf ( clear bit in a file register) - so to turn off the LED, you would use bcf PORTB,0. In the same way, we can use these instructions to set and clear RP0: bsf STATUS, 5 You saw above that RP0 is bit 5 of STATUS. However, thanks to the.INC file, the assembler knows that RP0 means 5 (find and open the.INC file to prove this to yourself). So we can write: bsf STATUS, RP0 Which is much easier to remember, and easier to understand when you revisit your code some time after you wrote it.
Incidentally, this same syntax applied before when we wrote btfss STATUS, Z - the Z was actually converted to a 2 by the assembler. Final programme: Right. We've covered a lot of ground here.
We've met a lot of new instructions, and concepts - especially in the last few sections. Programming a PIC processor is much easier if you have an understanding of the internal architecture, especially as it varies from the 'normal' computer systems that you might already seen. Von-Neumann Architecture: This, if you like, is the 'normal' way to build a computer system. The address and data buses are common for all memory access. Program-code and data exist in the same memory range, along with any memory-mapped peripherals. This is the most common architecture in use Pros:.
Pic Programming Tutorial For Beginners
Simplifies hardware (both circuit-design and layout) - routing multiple data-buses can be awkward on compact layouts. Easier to generate re-locatable code, which makes multi-tasking easier to implement. Perhaps not an issue here, but consider a complicated product such as a digital set-top-box. Cons:.
Instructions must be multiples of the data bus-width - can be inefficient. Variable number of cycles required for instructions. For example, an instruction that requires data from memory must wait at least another cycle before it can complete, whereas some instructions execute much faster. This can be a problem for time-critical applications. Harvard architecture: This architecture overcomes the memory bottle-neck by splitting the memory into instruction (code) and data areas.
These are accessed via separate data and address busses Pros:. Data and address busses can be different widths.
This means the programme memory word can be wide enough to incorporate an instruction and a literal (fixed data) in a single instruction. A built-in two-stage pipeline overlaps fetch and execution of instructions, meaning most instructions execute in a single clock cycle. Cons:. Slightly more confusing at first. Hardware is more complicated. Luckily, Microchip have taken care of that! To summarise, programme memory is different to data memory - in the PIC16FXX series, the programme memory is 14 bits wide, whereas the data memory is a more 'conventional' 8 bits wide.
Remember - PICs are essentially an 8-bit processors. We'll see much more about this later. Registers and Memory: Like any computer system, the PIC processor has a memory-map - as you can see, there are 256 different locations shown here - this suggests that 8 bits are required to address all the locations. The memory map has a 2-dimensional aspect - 7 bits will give you 0 to 127, moving you up and down the map, and the eighth bit will move you across the map, giving the total of 256 possible address locations.
This is called paging or banking. As you'll see later, this 8th bit is extremely important - it's called RP0 and is contained in the STATUS register. A question to ponder - you'll note that the STATUS register is available on both 'sides' of the map. Why is this essential? FFh (255) - Not implemented The important point is that Data memory contains a mixture of RAM (GPR's in Microchip-speak), special registers and peripherals. Not shown is the Working Register, or 'W'. This is equivalent to the Accumulator on other CPU’s.
Using Peripherals: Before you can use a peripheral, you must configure it. Predictably, this varies in complexity, but can be very straightforward.
For example: Port B - 8 bit wide bi-directional port: Individual bits of PORTB (address 05h) translate directly to physical pins on the IC. But, before the port can be used, you must program TRISB (the data-direction register). This tells the microcontroller whether the individual bits of PORTB are inputs or outputs. Example: Bits 0-3 are connected to LED's so we want them to be outputs. Bits 4-7 are connected to switches, so these need to be inputs.
Setting a bit in TRISB makes the corresponding bit of PORTB an input. Therefore, TRISB needs to be programmed with 11110000 Having done this, we can now read and write to PORTB. Writing 15 ('00001111' in binary) to PORTB will light all the LED's. Clearing PORTB (by writing '00000000') will extinguish them. Reading PORTB and masking the lower bits will reveal the state of the switches. Interrupts: These are a powerful way to ensure that a peripheral is able to demand the attention of the CPU immediately!
On receiving the Interrupt Request (IRQ), the following happens:. The current Program-Counter is stored on the stack. Program flow is diverted to the Interrupt Vector.
The users program (optionally) stores certain key variables, such as W, STATUS, etc. The source of the interrupt is determined (again, optionally) and the appropriate code can be executed. Key variables are restored, prior to. Ending the ISR and resuming normal program flow. Note: Red steps are automatically performed by the microcontroller. Some applications are entirely interrupt-driven, and do nothing until receiving an interrupt.
In this case, there's no need to perform the optional steps. The PIC16F84 has 4 interrupt sources:. External interrupt RB0/INT (bit 0 of PORTB).
PORTB change interrupt (bits 4-7 of PORTB). TMR0 overflow.
Data EEPROM write complete interrupt Typical examples include:. Infra-red receiver input. Keypad inputs (in conjunction with the internal PORTB weak pull-ups). Maintaining a real-time clock.


To allow the PIC to continue the main program flow while writing to the EEPROM memory. I feel that it is important to be aware of interrupts, and not to be frightened by them! Interrupts are essential to almost any real application, and are essential-learning! This simple overview of the PIC architecture will hopefully help your understanding during the next few Posts, where we start to write our first programme. Having experimented with different ideas, I arrived at a way of working that is quick and convenient. This page provides a step by step guide to configuring your PC to work with my chosen range of software.
Please note - I'm not necessarily saying that this is the best way to do this, but if you're in a hurry to get started, this will ensure your system is the same as mine and all of the following sections will work. Once you've gained confidence and experience, I hope you'll feel free to try different ways of working. If you discover a better way, please let me know.
In short, here's what we need to do:. Check the (modest) system requirements.
Install, the free assembler from Microchip (a half-meg download). Install, a free PIC programmer (another half-meg download). Install, the best ever text editor! (Just over a MB) 1. System requirements: For PIC programming, I use my workshop PC that has a minimal installation of Windows 98.
Because I rely on this machine, I deliberately keep it 'lean'. Despite being an old OS, it's absolutely fine - in fact, it's one of the quickest, most stable Windows machines I've ever used. Note that it doesn't connect to the Internet, so it doesn't need to run a virus checker or a firewall - this makes a big difference to the performance. It's a 333MHz Celeron with 256MB of RAM, and a fast 30GB hard drive. It reboots in less than a minute, so on the rare occasions that you see a GPF, it's no hardship to restart it. Keeping it free from XP and all the pretty but pointless visual widgets is the key to the performance. I've run all the applications very successfully on much slower machines (Pentium 1's with much less RAM).
The software is not demanding, and the machine spec really affects the way Windows runs more than anything else. You hardly need any disc space either. In general, it seems safest to stick to low-spec machines that run Windows 98 - a good use for old machines that have been obsoleted by Microsoft! Having said that, all of this should work just fine under XP but I haven't tested it yet!
I might expect problems from IC-Prog because of the serial port control. Also, some laptops don't provide the full 12 volts from their serial ports, so this might cause problems. Note that I've had problems getting the ConTEXT function keys to work with NT4, so this is best avoided. Install MPASM: You need the latest version of MPASM, the Microchip PIC Asse mbler. This can be found on the - just type 'MPASM' into the search box on the front page. At the time of writing, it's a 565KB download.
If you like, you can download MPLAB, which is their development environment that includes MPASM. Personally, I don't use MPLAB, but don't let that stop you. Recent versions of MPLAB are really quite good, and it includes a simulator which is occasionally useful. If you're on a dial-up connection, you may not want to do this as it's a 38MB download! (version 6.6) Installing it is just a case of following the instructions. Decide now which drive and folder you are planning to install it to, as you'll need to know this later.
For all sorts of complicated historic reasons (habit!), I installed it on my F: drive, in F: CAD PIC MPLAB (Yes, I did install MPLAB) The installation will create a program group, and you should have a shortcut to MPASMWIN (there is a DOS version of MPASM). Run this, and check the options: From memory, you shouldn't need to change too many of these, but make sure the List File box is ticked.
The 'Default' setting is appropriate for most options, because all of these can be specified in the source code that you'll write later. Install IC-Prog: Download IC-Prog from - it's a 550KB download. Decide now which drive and folder you are planning to install it to, as you'll need to know this later.
For all sorts of complicated historic reasons (habit!), I installed it on my F: drive, in F: CAD PIC PICProg (You have to create your own folder). You'll need to configure it to work with your programmer. The screenshot shows the options I set for my JDM programmer, but feel free to experiment. You'll note that I've selected the PIC16F877 - you have to set this before loading the.HEX file, because when you change device, it empties the buffer. If you are running this from ConTEXT (see below), you'll need to run up IC-Prog before and select the processor. Another option that I changed is to enable Verify during programming, which is in the Options dialogue. Otherwise, it will spend ages programming the device, and won't flag up a problem until it starts to verify.
A PIC16F877 has 8KB of programme memory so this takes a while, and is very frustrating! Before proceeding, you should ensure that you can write a.HEX file to a PIC, and read one back from the PIC.
Use the.HEX files discussed. Install ConTEXT: I discovered some years ago that ConTEXT is the best text editor for many applications, particularly PIC programming. I haven't seen a better option yet. We are going to install and configure ConTEXT to behave as our 'shell', or environment. Download the latest version from - note that this text refers to 0.x versions (0.97.5 at time of writing). For some time now, we've been anticipating the release of Version 1 which promises to be radically different, which I hope is a good thing. Should version 1 be available, the following instructions might not work.
The download is 1.18MB, so fairly dial-up friendly. Installation is a simple case of running the.EXE, and choosing which directory to install to. Onan 4500 commercial generator service manual. For all sorts of complicated historic reasons (also habit!), I installed it on my D: drive, in D: Program Files WinUtils ConTEXT (After installing Windows, I create Program Files on my D: drive and install all applications there, thus keeping the C: drive as clean as possible. This makes reformatting and rebuilding much easier) Next I place a shortcut to ConTEXT (and IC-Prog) on the quick-launch area of the taskbar.
I've never like the Start menu. Now, the fun begins. First, we need to install the correct Microchip PIC highlighter. My version is here, but feel free to make your own, as mine is a 'work in progress'.
Every few months I discover a new keyword that should be in the list! Alternatively you can download one from the ConTEXT site - I haven't tried this, so I can't comment on it. Either way, copy the appropriate.CHL file to the Highlighters directory within the ConTEXT programme directory and restart ConTEXT.
Please note that the following step might not be necessary with the most recent version of ConTEXT: Before this will work correctly, we need to tell ConTEXT not use the x86 Assembler with.ASM files. Open a file within ConTEXT - any sort of file, we just need to run ConTEXT with all the menus showing.
Free Programming Lessons For Beginners
Then, drop down the Tools menu and go to Set Highlighter. Choose Customize Types. And find x86 Assembler in the list.
Click on Edit, and change the asm that appears to something else. I just placed an underscore at the beginning. 'OK' that, and you should find it uses the correct custom highlighter for.ASM files. You might be wondering why I feel that syntax highlighting is so important. Apart from making the source code look nice, it performs an essential role for beginners - there are so many new instructions and keywords to learn that any help you get from the computer has got to be welcome. As you're typing in your source code, seeing that the instruction changes colour and is shown in bold is good positive feedback - you know you've just typed something that the assembler can understand!
Next, make sure you have a.ASM file to play with. It can be anything - we just need to have the file open in the editor, because we're going to customise the function keys. You're about to see why ConTEXT is so good! Drop down the Options menu and select Environment Options. In the dialogue box, click the Execute Keys tab - you should see a tree in a white box showing 'asm' as a branch, with F9 to F12 hanging from it. F9 - Assemble this program: First, click on F9, then click the button on the right of the Execute box. Browse to the folder you installed MPASM in, and select MPASMWIN.EXE - note that if you installed MPLAB, you'll find MPASM in a sub-folder called 'MCHIPTools' or similar.
This means that if you press F9 while editing a.ASM file, the Microchip Assembler will run. PIC's are a good microcontroller family to get started with.
As there are only 35 or so instructions to learn, programming them in assembly is relatively simple. That's good, because Microchips assembler is freely available from their. Alternatively, you can get use a C compiler or PicBasic which might appeal if you've never tried assembly language, but you have to pay money for them.
Actually programming or 'blowing' your code into a PIC is potentially as free as producing the code, although you might prefer to buy an inexpensive programmer because that should eliminate one of the several variables that can conspire against you at the beginning. There's plenty of designs on the web, complete with the software you need. I built the, a well-known device that is powered from the serial port.
To test it, it's worth downloading which contains 3 DOS executables that enable you to test the programmer, program a PIC and read the code in a PIC. This programmer works with most serially-programmable PIC's, although you need to build an adapter for any that are larger than 18 pin devices.
See the diagram on the JDM site which shows you how to place 8-pin devices I've redrawn the schematic in a form that makes more sense (well, to me, at least!). I've added a couple of LED's, and you might notice that I've wired D6 differently - simply because I didn't have the right zener at the time. The Veroboard prototype worked well enough, but as I had some spare real-estate on the PCB that I designed for the Olde 'Scope repair, the programmer took on a more professional appearance: One of the best midrange PICs for a beginner is the PIC16F84. It uses Flash technology for the program memory, and can be reprogrammed around 1000 times. These devices have 13 IO ports, and support interrupts, as well as having 64 bytes of non-volatile EEPROM memory that your program can use as permanent storage.
There are many variations on the theme, including PIC's with A-D converters. Once you've got to grips with the family, taking advantage of the extra features of the more advanced chips ought to be straightforward. The (slightly) bad news is that it takes a while to come to terms with the basics. For example, most people will be aware of the more common 'Von Neuman' architecture, where program and data memory are shared. PICs use the 'Harvard' architecture, where program memory is separate from data. This has a number of advantages, as we'll see later. Testing the programmer: Once I'd built the programmer, I wanted to programme a PIC.
Surprisingly, a quick web search failed to find anything simple enough, so I wrote a simple 4 bit counter which I managed to get working after much head-scratching. You can download the.HEX file here (you might need to use right-click, Save As). This simple program counts from 0 to 15.
To build it, you need a PIC16F84, 4 LED's and 4 resistors, and a crystal or resonator. The frequency of the crystal determines the count-rate - around 1 second with a 4MHz crystal. For the purposes of experimenting with PIC's, I'd recommend using a solderless prototyping board. The connections are very simple, as shown here: You might need to add 33pF capacitors from each crystal pin to ground, but I found that there's enough stray capacitance if you're using prototype board. The reset pin can be connected directly to +5V because the 16F84 has an on-chip reset circuit.
You'll need to have 4bit.HEX it in the same directory as PICPROG.EXE (contained in ). Then, at the DOS command line, type: picprog 4bit.hex 1 (assuming the programmer is connected to Com1.) In about 10 seconds, it's all done. Don't worry about the verify failure - this happens because the verify routine examines the whole chip contents, whereas the programme routine has only written to the locations specified in the.HEX file. Remove the PIC from the programmer and place it in your circuit. Apply power and watch those LEDs count binary. If nothing happens, double-check the polarity of the LED's and try to ensure that the clock is running - an oscilloscope is useful here.
Please note that if you're not confident using DOS commands, you may choose to use IC-Prog, the Windows application that I recomend you start out with. Details on the next page. On to the next Post configuring your PC to program PICs. What is a Microcontroller? Microcontrollers are used in many items of electronic equipment. They are not a new invention - back in the early 1980's, there was 'digital revolution' in the consumer-electronics market which fuelled their growth. For example, CD players required intelligent microprocessor control, and increasingly television sets were sold with remote control, digital tuning systems and teletext decoders.
Mechanical 'piano-key' controls seen on domestic video recorders were replaced with tactile 'soft-touch' logic-controlled buttons. While they have a very strong presence in the consumer market, they are also seen in broadcasting and industrial environments. Current microcontrollers are extremely powerful, and can run complex 'real-time' applications that are able to multi-task many different hardware functions while providing a nice user-interface for the engineer or operator. The Microchip 'PIC' family is a good range of microcontrollers to study. They are not too difficult for a beginner to learn, yet they are powerful enough to be genuinely useful. You'll find that you can replace complicated logic functions that require many standard TTL logic IC's with just one PIC.
Or, using just that one PIC, you could implement something that is easily beyond the capability of TTL logic. Real-world example - FM tuner: Starting at the very beginning, it is worth considering the difference between a microprocessor and a microcontroller. Consider the following example. This diagram shows the analogue signal path in a typical FM tuner - you might recognise most of the components shown here. But, most importantly, note the control connections that are required between the analogue hardware and the control circuitry. The phase-locked-loop (PLL) is controlled by a two-wire serial control bus. There are different standards in use, but one of the most common standards is called I2C (this stands for Inter-IC bus - a standard invented by Philips some 20 years ago) This particular PLL IC happens to have a standard TTL-compatible output to indicate if the PLL is locked and therefore 'happy'.
This is the easiest way to transfer 'status' information or commands from one piece of hardware to another, but in a more complex system this could be inefficient. Remember, the signals will require PCB space, cable and connectors. In this example we have another 3 TTL-compatible signals:.
A signal from the stereo decoder to inform the control system when we are receiving a stereo broadcast. A signal from the control system to tell the stereo decoder to operate in mono or stereo mode. A signal to mute the audio outputs (this would be used while tuning or during no-signal conditions to prevent a loud noise being heard). Finally, there is an analogue voltage from the FM demodulator which is used to provide a Received Signal Strength Indication (R.S.S.I.). This is used to provide a front panel display of the signal strength, and also to enable station-search operations. Obviously, this analogue voltage needs to be converted to digital using an ADC (analogue to digital converter) before the control system can use it.
Here's the required control hardware: If you have studied computing systems in the past, you might recognise the major blocks here. While the CPU is the heart of the system, in order to function it requires RAM, ROM and memory-mapped IO. The programme code required to make this otherwise useless collection of hardware act as an FM tuner is stored within the ROM, and the RAM contains all the variables needed to perform this task - such as the current frequency. The latter block, which could be formed with standard logic, is used to 'translate' an address in memory to physical IO lines on the circuit board. So, for example, to check the state of the 'PLL LOCKED' signal, the software will just read a particular memory location. To the CPU this looks no different to any address in the memory-map. But in reality, the memory-mapped IO will translate this to a 'real' signal.
In addition to the four IO lines from the analogue circuitry, the memory-mapped IO provides 'connectivity' to a number of different parts of the system: the ADC, I2C interface, the EEPROM memory (which might store your preset radio stations) and the user-interface formed by the keypad and display. In short, the memory-mapped IO forms a large part of the hardware in a typical microcontroller system. Finally, you'll notice some peripheral circuitry - the clock oscillator and reset generators should be familiar building blocks. The infra-red remote control signal is decoded with the help of an interrupt because of the precise timing requirements.
This is a large and complicated piece of hardware, which you won’t see this when you remove the lid on your hi-fi tuner. Instead, you will most likely see one large IC, which connects directly to the display, front panel controls and the analogue sections of the tuner. All of the blocks above can be integrated into one chip, and that IC is called a microcontroller.
On to the next Post - first steps.